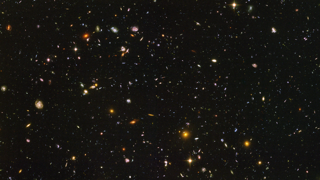
Space Telescopes
- 25th Jan 2024
- Author: Katie Holland
The first space telescope, the Orbiting Astronomical Observatory 2 (OAO-2), was launched into low Earth orbit in 1968 aboard an Atlas-Centaur rocket. For the next 4 years, OAO-2 beamed data back to scientists on Earth, including the first observations of astronomical objects in ultraviolet light.
Since then, dozens more telescopes have been sent into space, allowing us to learn more about the Universe around us than perhaps was ever thought possible. But why should we go to the effort of sending a telescope to space in the first place? And what is ultraviolet light?
Light and our Atmosphere
Here on Earth, we use telescopes to observe distant objects out in space. They allow us to capture stunning images of our night sky and have been used by astronomers to make new discoveries about our surrounding Universe. Most telescopes work by detecting the visible light released by an object – that’s the kind of light we can see with our eyes. However, there are actually lots of other types of light that can be detected by more advanced telescopes and observatories. We call the full range of these different types of light the electromagnetic spectrum. There are seven types in total: radio, microwaves, infrared, visible light, ultraviolet, X-rays and gamma-rays. The forms of light are grouped according to their wavelength, a property of the radiation. Radio waves have the longest wavelength and gamma-rays have the shortest. We use electromagnetic radiation for all types of things here on Earth, from taking medical X-ray images of our bodies to heating food in the microwave. It is also very useful to astronomers. By observing celestial bodies in these different types of light, we can learn new information and pull out new details that we may not have been able to see before.
This is where it becomes important to use space telescopes. As electromagnetic radiation comes towards Earth, it interacts with our atmosphere in ways that can distort our view of an object. The most common way we see this is through a process called refraction. As light passes through different substances, it is bent in different directions. This same effect happens as light travels through the different layers of Earth’s atmosphere. By the time the light reaches us on the ground, it has become so warped that the stars seem to twinkle. So stars don’t actually twinkle in space, they just appear to as we view them from Earth! Now imagine an astronomer trying to take accurate measurements of this star with their telescope – the twinkling is going to make that pretty difficult. If, instead, the astronomer could use a telescope placed above Earth’s atmosphere, they would be able to take much more detailed observations.
Additionally, the atmosphere can block out some types of electromagnetic radiation and prevent them from reaching the ground. This can sometimes be useful – for example, the ozone layer protects us from harmful ultraviolet radiation released by the Sun – but isn’t very helpful to astronomers trying to detect those types of light. Sending a telescope into outer space exposes it to the full electromagnetic spectrum, allowing it to gather more information about what it is trying to observe.
Space Telescopes
As of the time of writing, there are more than 30 active space telescopes, and even more that have ceased operating since their launch. Here, we will take a look at a handful of these telescopes covering different parts of the electromagnetic spectrum.
-
NASA Hubble
The Hubble Space Telescope is perhaps the most prolific observatory to have been sent into space. It was launched in April 1990 and had an expected mission lifetime of 15 years, which it has since far exceeded. Hubble continues to send data back to us to this day, including some of the most iconic space images ever produced. Hubble is primarily a visible light telescope, but it can make observations in some infrared and ultraviolet wavelengths too. During its 33 years of operation, Hubble has also made some groundbreaking discoveries, including helping to pinpoint the age of our Universe and the rate of its expansion. Hubble's low Earth orbit has allowed it to be visited by servicing missions that have extended its operational life. However, no telescope can work perfectly forever, especially since the space shuttles sent on these missions are no longer in use. Scientists estimate that Hubble should remain functional at least until the end of this decade, after which point it will be retired.
-
NASA James Webb
The James Webb Space Telescope, launched in December 2021, is often considered to be the scientific successor of Hubble. Webb was designed to observe light from more distant stars and galaxies, which emit in infrared. This wavelength of light can reveal hidden details of some nearer objects. Infrared is able to pierce through the gas and dust of galaxies and unveil what's going on underneath, which is why regions of space (for example, the Pillars of Creation in the above section) imaged by Webb seem to have more stars than when they're imaged by Hubble. Webb's most striking feature is it's large gold-plated primary mirror, which collects the light from the object being observed. The mirror is gold because this material happens to be particularly good at reflecting infrared light, improving the telescope's function. The mirror is also huge with a diameter of 6.6 metres, about the height of three telephone boxes stacked on top of each other. This allows the telescope to collect more light and detect dimmer objects. Astronomers hope that Webb could potentially continue to send us information for the next two decades, helping to uncover the origins galaxy formation in our Universe.
-
NASA Fermi
The Fermi Gamma-ray Space Telescope, formerly the Gamma-ray Large Area Space Telescope, was launched in June 2008 to study the highest energy wavelengths of the electromagnetic spectrum. This type of light can be emitted from many sources in space, including active galactic nuclei (the energetic cores of galaxies) and supernova remnants (the remains of stars that have exploded at the end of their lives). Fermi has detected some of the most violent explosions of gamma-rays, including a gamma-ray burst called GRB 221009A, which was measured from over two billion light years away. These explosions originate from the most energetic events observed in our Universe, such as the birth of a black hole. The telescope has detected over 5,000 other similar sources of gamma radiation. Fermi was initially expected to operate for five years, but the mission has been extended with no signs of stopping any time soon.
-
National Space Centre XMM-Newton
XMM-Newton, launched in December 1999, is designed to detect hot X-ray sources in the Universe. This includes the study of black holes and hot gas present in regions of galaxy formation. The XMM part of its name comes from its X-ray Multi-Mirror design. XMM-Newton is quite unique in that the telescope is actually three telescopes bundled together! This allows it to collect more data than any X-ray telescope had before it. The model we have here at the National Space Centre is to 1:4 scale, meaning it's a quarter of the size of the real thing. The total length of the telescope is around ten metres, the same as a London bus. The telescope has been used to identify various parameters about black holes, including their mass, their rotational speed and the light they emit.
-
ESA Euclid
Euclid, like Hubble, is an optical telescope, meaning it makes observations in visible light. However, Euclid is different to some other space telescopes in that it isn't interested in finer details. Instead, Euclid is designed to make broad observations, ultimately aiming to image one third of the sky. Euclid will measure light detected billions of years ago, producing a 3D map of the sky with time as the third dimension. The telescope will analyse how billions of galaxies interact with and move apart from each other. On this scale, larger structures will become clearer and scientists will be able to study the presence of dark matter and dark energy in the Universe. Euclid's first five images were released in November 2023, just four months after its launch. These images offer a snapshot of the kind of results we can expect to see from the mission over the coming six years.
-
Space Dynamics Laboratory/Allison Bills SunRISE
SunRISE is an upcoming mission that could launch as early as 2024. Six small satellites, each around the size of a cereal box, will be sent to study the Sun's radio emissions. This makes the mission quite unique, as radio space telescopes are considerably rarer than those studying other parts of the electromagnetic spectrum. This is because most radio wavelengths can penetrate Earth's atmosphere and reach the ground. The exception to this are the long radio wavelengths that SunRISE will measure. The spacecraft will operate within 10 kilometres of each other and use a process called interferometry to communicate with each other and act as a single, more powerful telescope. The mission will continuously study solar storms, with the aim of allowing for better space weather prediction.
Space telescopes have revolutionised our understanding of the Universe. They've turned points of light (and even things that are out of sight) into magnificent views of planets in our 'backyard' and galaxies in the distant depths of the cosmos.
It's exciting to think what the next generation of space telescopes will open our eye to!
Full references/credit:
(Banner Image) The ‘Hubble Ultra Deep Field,’ a view of nearly 10,000 galaxies imaged by the Hubble Space Telescope. Credit: NASA, ESA, and S. Beckwith (STScI) and the HUDF Team
(1a) ESA space telescopes across the electromagnetic spectrum. Credit: ESA
(1b) Atmospheric effects on different parts of the electromagnetic spectrum. Some parts make it further through the atmosphere than others. Credit: Adapted from STScI, JHU, NASA
(2) A comparison of the Pillars of Creation imaged by the Hubble Space Telescope in visible light (left) and infrared light (right). Credit: NASA, ESA, Hubble Heritage Project (STScI, AURA)
(3) The Hubble Space Telescope in orbit above Earth. Credit: NASA
(4) The James Webb Space Telescope with its mirrors fully deployed in a 2020 pre-launch test. Credit: NASA
(5) An illustration of the Fermi Space Telescope. Credit: NASA
(6) The 1:4 scale model of XMM-Newton on display at the National Space Centre. Credit: National Space Centre
(7) An artist's impression of the Euclid telescope in space. Credit: ESA
(8) The six SmallSats developed for the SunRISE mission. Credit: Space Dynamics Laboratory/Allison Bills
(9) Saturn imaged by the Hubble Space Telescope in June 2019. Credit: NASA, ESA, A. Simon (Goddard Space Flight Center), and M.H. Wong (University of California, Berkeley)
(10) The Carina Nebula imaged in infrared light by the James Webb Space Telescope. Credit: NASA, ESA, CSA, and STScI
(11) The Hidden Galaxy imaged by Euclid. Credit: ESA